Upgrade of the TPC Readout (during LS2)
The upgrade of the TPC aimed at an increase of the data-recording rate for Pb-Pb interactions by a factor of about 100. The MWPC readout chambers were replaced with Gas Electron Multiplier (GEM) detectors, and the new front-end electronics enable continuous readout.
The expected lead-lead collision rate during LHC Run3 (2022 onwards) is 50 kHz. This corresponds, on average, to a collision every 20 μs. In the ALICE TPC, a 90 m3 gas volume where ionization electrons take 100 μs to drift the full 2.5 m distance to the Readout Chambers, the equivalent of 5 events will overlap, which suggests that in order to record the information of all charged particles of all collisions, a continuous readout technique should be used.
This poses two severe problems for the Multi Wire Proportional Chambers used for the readout of the TPC during LHC Run1 and Run2. These chambers are composed of an anode wire grid, where the electron amplification occurs, sandwiched between a cathode wire grid and a flat pad plane, where the signals are read out. But in addition, on top of this structure there is another wire grid, called the gating grid, which makes it possible for the detector to perform at high event rates and multiplicities. Introduced in the LEP era (ALEPH, DELPHI), the gating grid allows one to prevent electrons from non-triggered events to reach the amplification region, by applying an alternating voltage on its wires. Upon a trigger, the gating grid is quickly switched to a flat potential thus allowing ionization electrons of this particular event to reach the anode wires and induce a signal onto the pads. Now, the crucial role of the gating grid is to close itself just after all electrons from our event have reached the anode grid, such as to trap the positive ions produced in the avalanches and prevent that they invade the drift volume. In the same way, charge from non-triggered events is never amplified and thus no extra positive ions are produced. This mechanism allows one to keep the drift volume relatively clean of slow drifting ions (160 ms full drift time) which otherwise would build up a considerable space-charge density and lead to important distortions of the electric drift field. For example, if the current TPC would be run, without switching the gating grid, at 50 kHz Pb-Pb, tracks would appear distorted by as much as 1 m. But 1 m distortions are obviously too much to correct for. If we were to use the gating grid, the maximum trigger rate would be determined by the 100 μs for the drift of the electrons (gating grid open) plus another 180 μs for the corresponding ions to reach it (gating grid closed); this is about 3 kHz, much lower than the 50 kHz the LHC is expected to provide. So a gating technique is not possible.
Furthermore, the amount of charge reaching the anode wires would lead to the saturation of the amplification field in their vicinity, thus affecting the uniformity of the gas gain. Now, remember here that one of the functions of the ALICE TPC is particle identification through measurement of the specific energy loss of all charged particles; but if the gain is modified by fluctuating space-charge in the amplification region, the dE/dx determination will be seriously affected. So wire chambers altogether won’t do the job.
Looking for alternative solutions for the readout chambers, an obvious choice e micro-pattern gaseous detectors, GEMs. These Gas Electron Multipliers, foils with lots of tiny holes introduced by F. Sauli in the 90’s, are capable of coping with the rates and multiplicities expected and provide ‘intrinsic ion blocking’. However, standard configurations of triple GEMs do not provide sufficient ion blocking. We define the Ion Back-Flow (IBF) as the number of positive ions, after amplification, escaping back into the drift volume per initial primary electron. In order to keep the track reconstruction distortion to a bearable level, of order of 10 cm, the IBF from a GEM structure should be 1 % or below. Standard triple GEMs achieve about 5%. After an intensive R&D programme, an IBF below 1% has indeed been achieved by using non-standard configurations of stacks of 4 GEMs, with hole pitches different from standard, and with voltages and fields different from standard. It turns out that the minimization of IBF enters in competition with the energy resolution, i.e. the precious dE/dx performance, and by careful optimization of the GEM structure a good compromise has been reached.
But what about the stability of such an arrangement? GEMs have been optimized for years in order to be robust against discharges. Although we have departed substantially from the standard configuration, it turns out that the sharing of the gain between four, rather than three, GEMs provide, at our odd fields, the same discharge probability (about 10-8 for alpha particles) as the standard device. It should be noted here that, for various reasons, the operating gas of the upgraded TPC is Ne-CO2-N2 (90-10-5), where the addition of N2 has proven to strengthen the stability.
So we think we have a concept that guarantees charged-particle momentum determination and excellent particle identification through dE/dx. And we keep the beautiful field cage of the TPC.
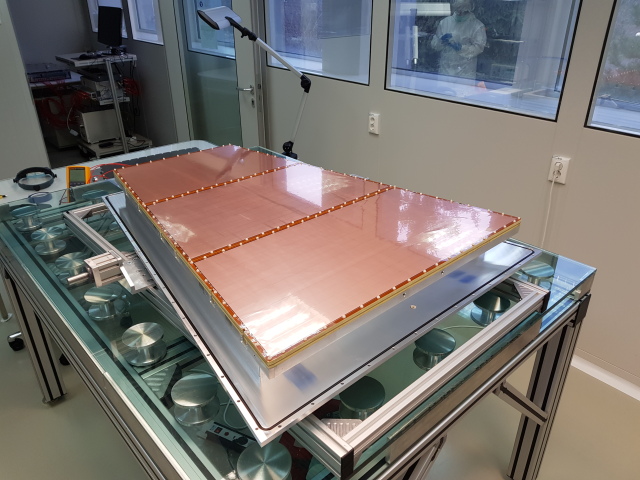
TPC readout chamber with GEM foils during assembly