Time Projection Chamber (Run 1 and Run 2)
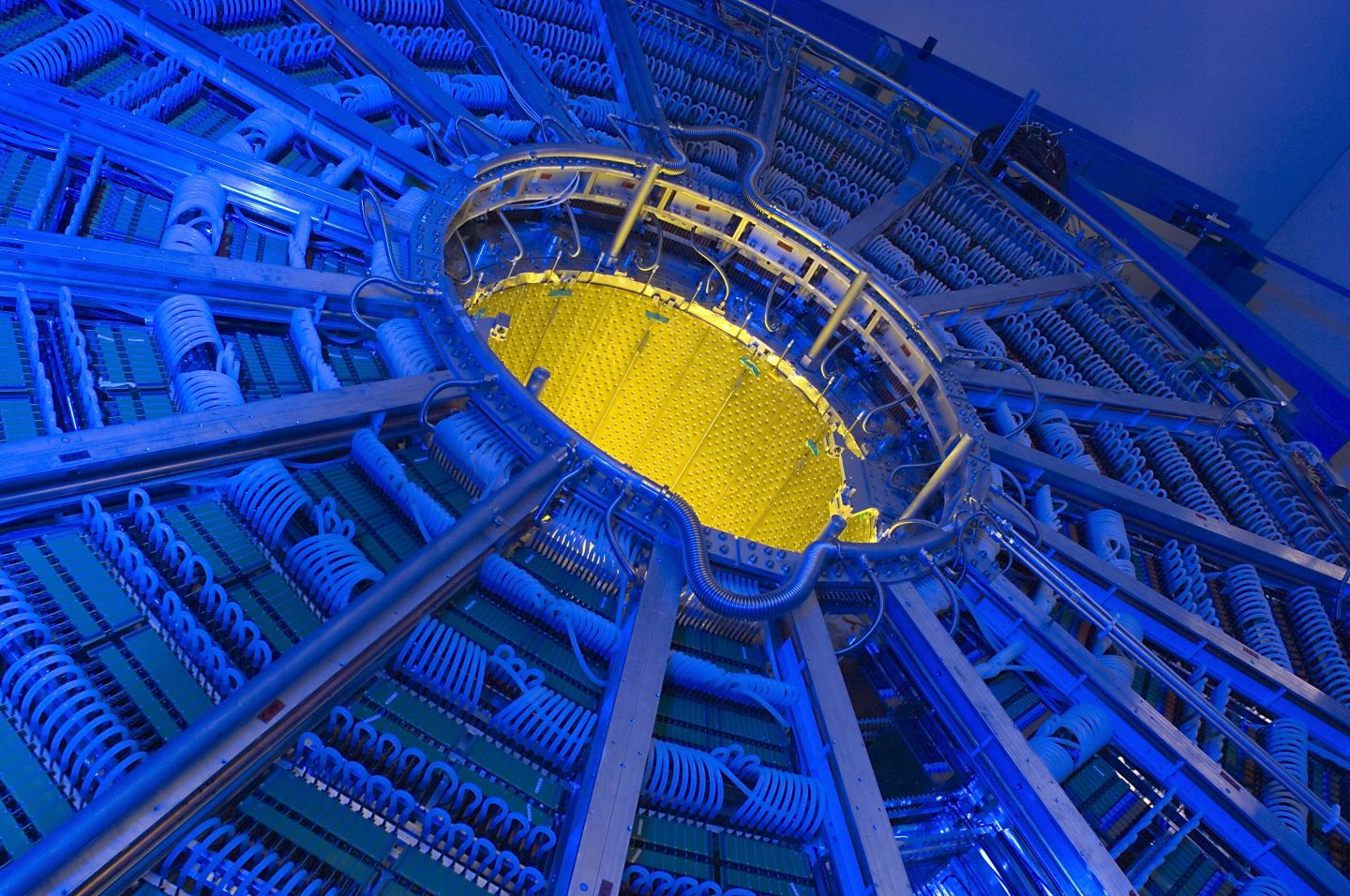
TPC for Run 1 and Run 2:
The ALICE Time Projection Chamber (TPC) is a large cylindrical volume filled with gas. Charged particles crossing the TPC ionize the gas atoms along their path, liberating electrons that drift towards the readout chambers covering the end plates of the cylinder. The signals read out by the 557,568 pads of these chambers provide 3-D information of the trajectory of the particles and measure their energy loss.
The ALICE Time Projection Chamber (TPC) is the main tracking detector of the central barrel. Through the study of hadronic observables, the TPC provides information on the flavour composition of the collision fireball and on its space-time extent at freeze-out. In conjunction with the ALICE Transition Radiation Detector (TRD) and the Inner Tracking System (ITS) it is used to study vector meson resonances, charm, and beauty through the measurement of leptonic observables.
A bit more detail:
The ALICE TPC is a 90m3 cylinder filled with gas and divided in two drift regions by the central electrode located at its axial centre. The field cage secures the uniform electric field along the z-axis.
Charged particles traversing the TPC volume ionise the gas along their path, liberating electrons that drift towards the end plates of the cylinder. The necessary signal amplification is provided through an avalanche effect in the vicinity of the anode wires strung in the readout.
Moving from the anode wire towards the surrounding electrodes, the positive ions created in the avalanche induce a positive current signal on the pad plane. This current signal, which is characterised by a fast rise time (less than 1 ns) and a long tail with a rather complex shape, carries a charge that, for the minimum ionising particle, is typically 5-10 fC. The readout of the signal is done by the 557.568 pads that form the cathode plane of the multi-wire proportional chambers located at the TPC end plates.
Field Cage
The design of the field cage of the ALICE TPC is based on a novel construction principle to adapt the detector to the specific running conditions with heavy ion collisions at the LHC. The high particle densities make it necessary that the field cage keeps instrumental (systematic) errors at a minimum in order not to impair the sensitive pattern recognition and resolution capabilities of the detector as a whole. Although a classical cylindrical geometry - optimum for colliding beam experiments - was chosen the other features of the device differ largely from any other field cage.
Separated by the central HV electrode, the field cage has two detection volumes with an inner/outer diameter of 1.2/5 m and a drift length of 2.5 m each. The total sensitive detector volume is 88 m3, filled with a gas mixture of Ar-CO2 (88-12). With a drift field of 400 V/cm, this gas represents the optimum in terms of charge transport (velocity and diffusion), signal amplification (order 104) and transparency for traversing particles. Hence, the field cage has to sustain a maximum potential of 100 kV at its central electrode. Consequently and in line with the requirements of ALICE, the field cage vessels were built from light materials, yet with sufficient mechanical rigidity for such a large apparatus. A composite honeycomb sandwich structure was thus chosen for its favorable stability/mass ratio. Material of aerospace quality has been used such as aramide-based honeycomb cores (Nomex) and foils of Tedlar. A principal element of our design philosophy is to 'contain' the actual field cage volume by a protective CO2 gas envelope provided by two additional cylinders called the inner and outer containment vessels. Containment of the drift volume is essential for personnel and operational safety. It also allows a substantial reduction in material traversed by particles. Another unique feature of the field cage is its internal potential defining system designed to provide a highly uniform electric field with radial distortions of no more than one part in several thousands. The entire potential strip network is suspended from 18 support rods mounted equidistantly over 360 degrees, 31 mm away from the cylinder walls. Although this system compromises physical acceptance it benefits from a substantial improvement in field uniformity due to the absence of charges accumulated between strips if glued on an insulating surface.
Readout chambers (LHC Run 1 and Run 2)
The ALICE TPC readout chambers were specially designed to cope with the high track density expected in heavy ion collisions at LHC. The pad size (granularity) of the inner chambers, i.e. those closest to the beam-beam interaction diamond, has been optimized (7 x 4.5 mm2) to the point that a signal induced in the pads after amplification at the anode proportional wires is just visible above the electronic noise (S/N>20).
Detail of the pad side of a readout chamber showing the segmentation into small pads, the three wire grids (anode, cathode, gating) and their contact strip.
This requires a very careful design of the front-end electronics to minimise the noise there, as well as a very high gas amplification (>several thousands). The high gas amplification implies copiously produced positive ions which, if not blocked by a gating grid, would distort the drift field significantly.
Thus the electrostatics of the gating grid, in particular at the chamber boundaries, had to be carefully designed to keep the ion feed back below the 10-4 level. After all optimisation steps it was nonetheless not a priori certain whether the chambers would work stably in a high track density environment. The chambers were subjected to secondaries from heavy beams mocking up LHC-conditions. These tests, as well as long term stability investigations with radioactive sources, have shown that the chambers are well suited to work in the ALICE environment.
Front-end Electronics
The signals from the pads are passed via flexible Kapton cables to 4356 front-end cards located some 10 cm away from the pad plane. In the front-end card a custom-made charge sensitive shaping amplifier transforms the charge induced in the pads into a differential semigaussian signal that is fed to the input of the ALTRO chip.
Each ALTRO contains 16 channels that digitise and process the input signals. Upon arrival of a first level trigger, the data stream is stored in a memory. When the second level trigger (accept or reject) is received, the latest event data stream is either frozen in the data memory, until its complete readout takes place, or discarded. The readout takes place, at a speed of up to 300 MB/s, through a 40-bit-wide backplane bus linking the front end cards to the readout controller unit. The ALTRO chip is a mixed-signal custom integrated circuit designed to be one of the building blocks of the front-end electronics for the ALICE TPC. In one single chip, the analogue signals from 16 channels are digitised, processed, compressed and stored in a memory ready for readout. The Analogue-to-Digital converters embedded in the chip have a 10-bit dynamic range and a maximum sampling rate of 40 MHz.
Click here to read the Technical Design Report for the TPC
Performance
The TPC shows excellent performance. The straightforward pattern recognition (continuous tracks) make TPCs the perfect choice for high-multiplicity environments, such as in heavy-ion collisions, where thousands of particles have to be tracked simultaneously. The picture shows an event display of a peripheral PbPb collision (with not too many particle tracks for best viewing) recorded in November 2010 (first PbPb collisions provided by the LHC).
PID in the TPC is based on simultaneous measurement of momentum and ionisation strength: for a particle with a given momentum the ionization depends only on its charge and mass, which are the parameters needed for PID. Inside the ALICE TPC, the ionization strength of all tracks is sampled up to 159 times, resulting in a resolution of the ionization measurement as good as 5%. The figure below shows exemplarily the TPC particle identification (PID) performance.
In the figure the functional dependence of the ionization strength on the momentum is shown as lines together with data. The particle separation capabilities are obvious: especially at low momenta the different particle families are well separated.
The described PID methods allows also the observation of rare antinuclei (with negative charge z >1). In the shown data sample, they were enhanced by the use of an offline trigger, which includes the PID information of the ALICE Time of Flight system. In this way, ten anti-4He nuclei were identified.
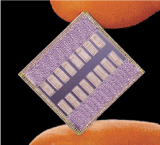
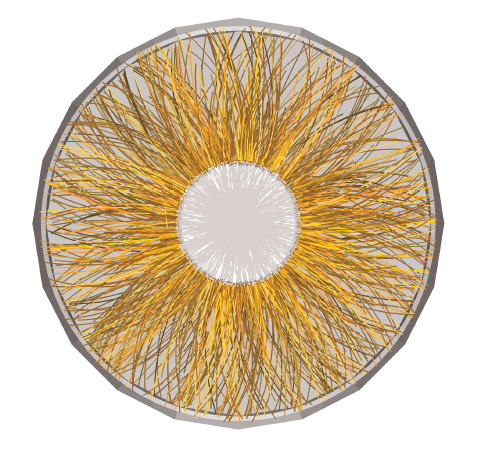
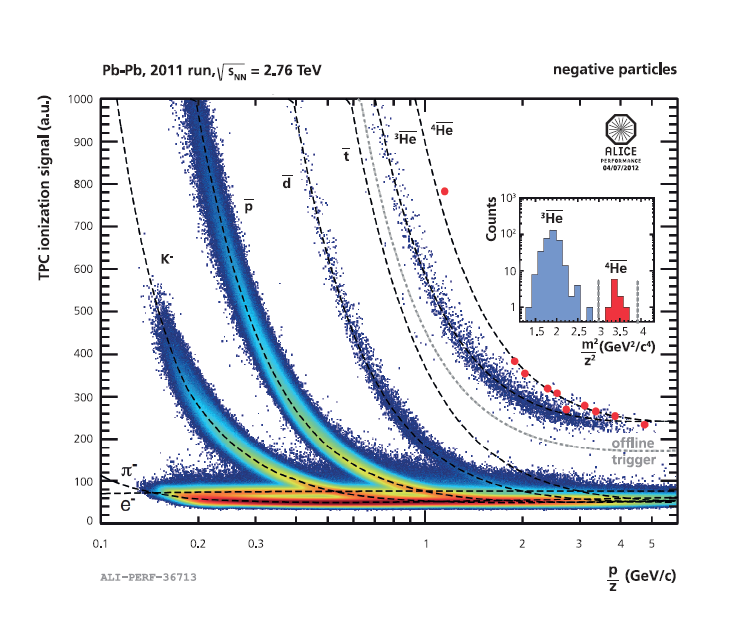
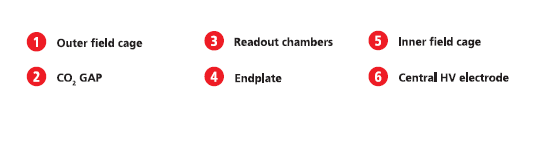